In the IMPACTS project we study the impact of impurities in captured CO2 on transport and storage. Questions we would like to answer are like these: what is the relation between the quality (level of impurities) of CO2 and the cost of safe transport and storage? Suppose we save some money and capture less pure CO2 (if there is such a relation), would that be offset by higher cost for transport and storage because of, for example, corrosion prevention measures? Can we optimize the cost of the entire CCS chain as a function of the quality of the CO2?
- Guest bloggers: Leen van der Ham, Filip Neele, TNO, The Netherlands. Blog originally published on the SINTEF Blog Sep. 11. 2015
Such questions are relevant for developers of CO2 transport and storage installations, as they will need to minimize cost and be prepared for potential future changes in the composition of the CO2. The IMPACTS project aims to study such relations for the impurities that can be expected to be present in captured CO2.
As a first step in the project, we had to find out what can be found in CO2, other than CO2 itself.
We had to decide which of today’s CO2 emission sources could be tomorrow’s capture plants, and how the CO2 would be extracted from the flue gases. In other words, we had to create an inventory of the impurities in captured CO2 from relevant emission sources, using state-of-the-art capture technologies.
This would define a range of impurities that can be expected in the first wave of CCS systems and would be leading the choice of impurities to study in the IMPACTS project. At the same time, this would also show the range in concentration of impurities that is relevant for the measurements and simulations in our project.
To create the inventory, we set up a number of combinations of CO2 sources and capture technologies. Then, for each combination, the composition of the captured CO2 was predicted, based on the composition of the flue gases and the capture technology. The final step was to select those combinations that produced extreme levels of impurities.
CO2 sources considered
According to the IEA, about two-fifths of the worldwide CO2 emission are related to electricity and heat generation, while other industries account for another one-fifth (IEA, 2012). Electricity generation is mainly done in gas- or coal-fired power plants. So, the sources related to power generation considered in IMPACTS are:
- Gas-fired power plants, and
- Coal-fired power plants.
Other important CO2 producing industries are:
- The steel and iron industry (31%)
- The cement industry (27%)
- Refineries (10%)
- High-purity CO2 sources like natural and synthetic gas processing (7%) (IEA, 2011)
Sources that were not included are for example the co-firing of biomass in power plants and other biomass conversion processes. Biomass is often considered a CO2 neutral energy source. Biomass conversion processes were therefore not listed among the CO2 producing industries.
CO2 capture technologies considered
The most common CO2 capture technologies have been divided in three categories in this project, based on the main separation task that is performed in order to obtain the purified CO2 stream.
- Post-combustion capture. The first capture category is based on the separation of a CO2 – N2 mixture at an atmospheric pressure, which is typically formed when combusting a fossil fuel in the presence of air. Common post-combustion capture systems are absorption processes based on amines or chilled ammonia.
- Pre-combustion capture. The second capture category is based on the separation of a CO2 – hydrocarbon mixture (HC) at an elevated pressure. Examples of the hydrocarbons that can be involved are H2, CH4, C2+, and CO, originating from coal gasification or from natural gas sources. Common pre-combustion capture systems are absorption processes based on physical solvents like methanol or dimethyl ethers of polyethylene glycol (DMPEG, Selexol).
- Oxyfuel combustion. The third capture category is based on the separation of an O2 – N2 mixture. By combusting a fossil fuel in the absence of N2, also known as oxyfuel combustion, the post-combustion CO2 – N2 separation can be prevented. But in order to do so, O2 must first be purified from air. This is typically done in a cryogenic air separation unit or in a chemical looping process.
The CO2 impurities inventory
Based on the six emission source types and the three capture technologies, we selected those combinations that produced the highest levels of impurities in the captured CO2 stream. The table below shows the results.
The EU CCS Directive requires that the captured CO2 consists “overwhelmingly” of CO2. This vague definition is often translated into a minimum required CO2 concentration of 95%. All cases produce CO2 with a concentration above this level, with the exception of coal-fired oxyfuel combustion. In a complex CCS infrastructure, CO2 from such a source could be acceptable, by mixing with CO2 from other sources. No water concentration is defined in the table, because it is mainly determined by the choice of dehydration specification.
The IMPACTS project uses this starting point to model the relation between impurity levels and CCS system design and performance. More information about the table and the framework for assessing the impact of impurities on CCS systems can be found in reports D1.1.2 and D1.1.3.
The IMPACTS project team is currently working to define various relations between levels of different impurities and the performance or design of CO2 transport and storage systems. This includes such effects as corrosion, the relation between CO2 composition and injection and storage, effects of impurities on external safety of pipelines. The work is based on measurements of the fundamental properties of CO2 mixtures, also performed within the IMPACTS project.
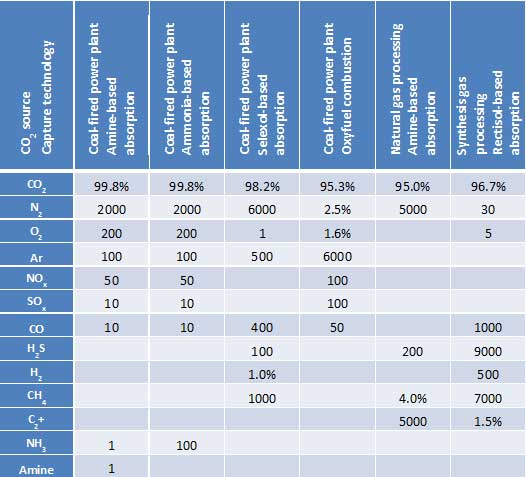
Comments
No comments yet. Be the first to comment!