Maximising impact from research is an important task for the Norwegian CCS Research Centre (NCCS). As such, the potential impact should be monitored to help ensure research effort is directed in the most effective way.
In 2019, an NCCS study looked at four of the research tasks to assess the potential impact of the research and expected innovations if the research were to be successful. The team hopes to do the same for the other research tasks during 2020.
Measuring the impact of research and innovations
Research within CO2 capture, transport and storage is multi-disciplinary and covers a wide range of topics. Consequently, the outcomes have different characteristics with respect to type of innovation, maturity and applicability in the CCS chain.
Learn more about CCS
Join our newsletter to stay updated with all the latest research results and news from NCCS: The Norwegian CCS Research Centre.
The innovations covered in this study mainly fall into four categories: new technologies, models and calculation tools, new methods, and new standards and guidelines.
Impact can be measured in several ways: reduced emissions, economic impact (increased value creation, lower costs), better decision making, saved energy, industrial potential, and more.
Here is a summary of the findings – but read on for the detail:
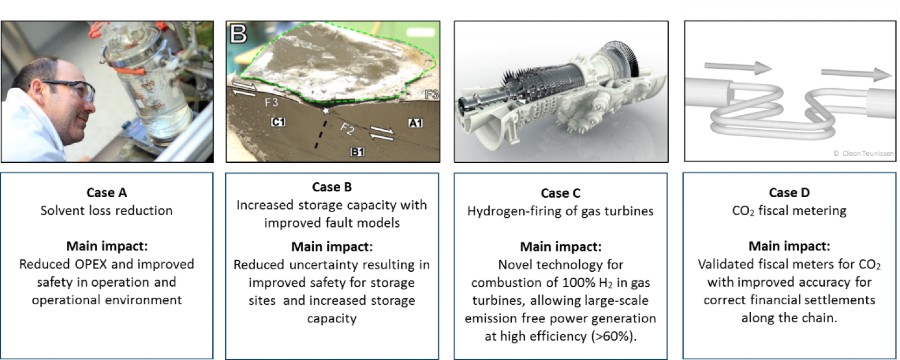
Designing a reference system
NCCS originally outlined two CCS deployment cases (DCs) to help structure and align its research, and to support the ambition to overcome critical barriers and accelerate CCS deployment. These DCs were used to brainstorm potential R&D barriers for short-term CCS deployment in Norway with smaller volumes of CO2 (DC1) and for large-scale deployment of CCS throughout Europe with storage in the North Sea (DC2).
For this NCCS impact study, a third case was defined to serve as a solid basis for the analysis: NCCS DC2030 – Unfolding CCS in Europe. It incorporates all CCS projects implemented and under construction in Europe, along with those planned to be operating by 2030. It includes industry sources, power generation, natural gas processing and H2 production. A combination of ship and pipeline transport of CO2 to aquifers and depleted gas fields ensures flexibility. Capacity in 2030 is estimated to 15-20 Mt/year, with ambition to increase to >40 Mt/year after 2030.
Now let’s take a look in more detail at the potential impact of the four NCCS tasks chosen for the initial study in more detail:
Case A: Solvent loss reduction (Task 2)
The challenge: During chemical absorption, CO2 present in a flue gas is absorbed and chemically bound to a solvent. The reaction is reversed during solvent regeneration and the solvent is reused to absorb CO2. A tiny amount of the solvent will react with other compounds in the flue gas forming compounds that cannot be regenerated. Over time, these reactions lead to loss of capture efficiency and could cause problems as corrosion, fouling, foaming and increased emissions.
The innovations: The work in NCCS investigates several mitigation methods including:
- Identification of solvents with higher chemical stability to minimize solvent loss
- Mitigation technologies, like removal of oxygen from the solvent as these components will enhance degradation
- Better understanding the degradation and corrosion mechanisms, allows development of innovative, new technologies to tackle the challenges
Base case for illustration: 2MTPA CO2 captured from 2 CGCC power plants using 30% MEA-based solvent technology. With the MEA cost of €2/kg and solvent degradation rate of 2 kg MEA/ton CO2, the cost of solvent loss is €8 M/year.
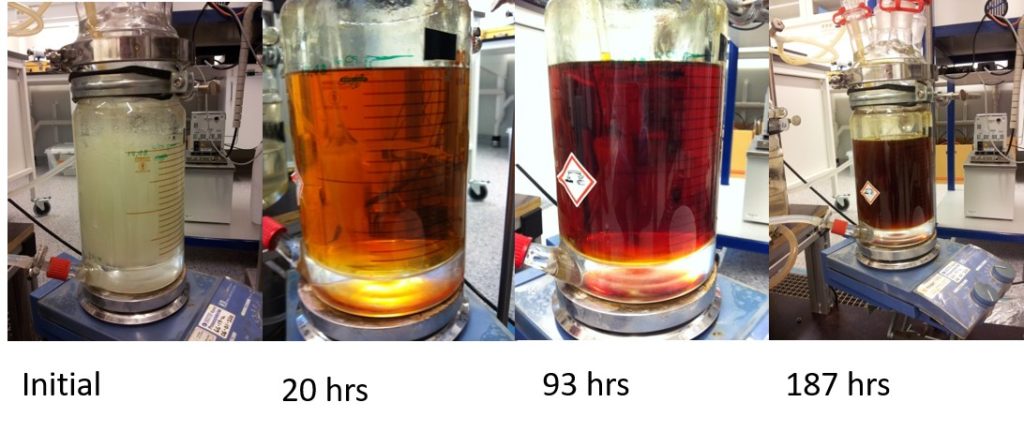
Potential impact: Implementing NCCS innovations for oxygen and iron removal will result in up to a 50% lower degradation of MEA based solvent. By reducing the need to replace active solvent components, the potential savings could reach €4 M/year.
In addition, fundamental knowledge developed in Task 2 on the structure–degradation relationship for various amines would enable selecting a stable solvent at the early stage of solvent development based on predicted degradation behaviour.
Case B: Increased storage capacity with improved fault models (Task 9)
The challenge: Implementation of large-scale CO2 storage will require utilization of a wide range of storage reservoirs including faulted reservoirs with structural traps. The sealing properties of faults are challenging to predict, and conservative estimates and high uncertainty may limit the total injection volume or even disqualify a storage site. Existing industrial models have limitations when addressing fault risk related to CO2 injection in faulted aquifers.
The innovation: The development of an improved fault derisking framework that includes dynamic pressure changes related to CO2 injection and addressing along-fault fluid migration is a main ambition of NCCS Task 9. Such framework can:
- Reduce uncertainty related to fault properties
- Increase confidence in site integrity and confinement
- Enable qualification of increased storage capacity.
Potential impact: The development of the Horda Platform area for CO2 injection showed that high uncertainty in existing fault seal prediction models for shallow, fault-bound aquifers like Smeaheia, limits the capacity and provides major obstacle for site qualification. The Norwegian CO2 storage atlas (NPD, 2012) indicates around 40 GT storage capacity in Norwegian North Sea aquifers. The effective volumes found suitable for safe and long-term storage during technical maturation may be as low as 10% of the estimated capacity.

For the Norwegian North Sea this gives a suitable safe capacity around 4 Gt. Assuming this volume could be increased with roughly 10% if risk related to fault sealing is reduced, a total 400 Mt increase in storage capacity can be estimated, enabling 20 years of storing 20 Mt CO2 per year (NCCS DC2030). Improved fault seal models and reduced uncertainty is a necessary, although not sufficient, step towards qualification of additional storage capacity.
Digital drill core models: Characterising and storing samples from Svalbard
Case C: Hydrogen-firing of gas turbines (Task 5)
The challenge: Hydrogen can be used for clean and highly efficient power generation with minimal CO2 footprint. Improved fuel flexibility of gas turbine combustion system is desirable, e.g. combustion of 100% H2 without the need of fuel diluents as N2 or steam injection. Known challenges for H2 combustion are:
- Flashback (off-design flame displacement): higher risk due to higher flame speed
- Autoignition (off-design early ignition): higher risk due to lower ignition delay time
- Combustion dynamics (thermo-acoustic instabilities): well-known thermo-acoustic amplitude level and frequencies for natural gas combustion are modified by hydrogen addition
- NOx emissions: due to higher flame reactivity, temperature and different structure/stabilization.
The innovation: Combustion technology development leads to potentially novel solutions to allow retrofit of existing gas turbines for combustion of 100% H2:
- H2-rich fuel injection system design
- Combustion chamber design
- Expertise in H2 combustion control
Potential impact
- Efficient large-scale power generation based on H2 from natural gas with CCS
- Compared to available technology the research aims to enable combustion of 100% H2, saving fuel costs by not needing diluents as N2, usually at a ratio of 10kg N2 per kg H2 to be combusted.
- Hence, achievement of carbon-free power generation that is both clean (low NOx) and thermodynamically efficient (>60%), at large scale (>1000 MW).
- Bridge H2 production from natural gas w/CCS and H2 from renewable sources, at large scale.
Reduced fuel costs are related to savings due to higher overall plant efficiency for the dilution-free advanced staging combustion system and estimated to 39 M€/year. The cumulative yearly H2 fuel cost for conventional N2-diluted H2 combustion system is 544 M€, while the cumulative yearly H2 fuel cost for pure H2 combustion system with advanced staging is 505 M€.

There will be considerable additional savings because of unnecessary process equipment for diluent (nitrogen or steam) preparation and reduced emissions as the CO2 avoided, if H2 is produced without CO2 emissions, of 2.1 Mt/year.
Base case for illustration: Baseline NG-fired CCGT: 750 MWe @ 60% efficiency. Key assumptions:
- 10 kg N2 pr kg H2 are the dilution requirements in conventional non-premixed systems (overall plant efficiency loss 4%)
- Firing temperature reduction in premixed systems with advanced staging (sequential/reheat) results in 1% overall plant efficiency loss
- H2 cost is 1,5 Euro/kg and cumulative yearly energy production target is 6.24 TWh
Case D: Fiscal metering and CCS fluid property research (Task 8)
The challenge: The European Union Emissions Trading System (EU ETS) is a pan-European system for trading greenhouse gas emission allowances. To be able to report avoided CO2 emissions through CO2 capture and storage in the EU ETS, traceable metering of CO2 is critical, and hence a business driver for CCS. In addition, custody transfer in CCS needs metering. Currently, no fiscal flow meter technology has been verified at relevant flow rates and conditions for large-scale CO2 management.
The innovation: The ongoing research in NCCS on fluid properties of CO2, modelling as well as targeted experimental investigations, and design of an industrial scale test centre aims for fiscal metering technology that can ensure traceable measurements for CO2 with quantified uncertainties. This can enable qualification of fiscal flow meters for CCS.
Potential impact: Qualified fiscal metering technology and procedures will make CCS relevant for EU ETS. For instance, those who have implemented CO2 capture can avoid buying allowances to emit CO2 (EUAs) under the EU ETS or sell EUAs they already have. With an increasing number of CCS projects and increasing costs of emission allowances, the total value of allowances will grow significantly.
For instance, in the Norwegian full-scale project the conservatively estimated annual value of CO2 traded under ETS can amount to 20 M€/year (800 k tonne CO2/year using today’s ETS carbon price of 25€/tonne CO2). For NCCS DC2030, the annual value of CO2 traded is 700 M€/year (20 mill. tonne CO2 /year using the ETS carbon prices projected in “EU Reference scenario 2016”; ca 35 €/tonne CO2).
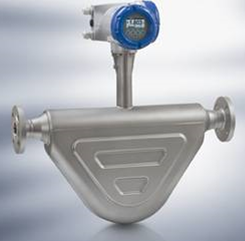
NCCS aims to enable verification and validation of commercially available fiscal meters for measurements of CO2 flow, e.g. from a capture site, certifying that the uncertainty of the stored CO2 mass is below ETS requirement, currently at 2.5 %. This will qualify the company for release of EUAs. In addition, innovations in NCCS will improve the accuracy of CO2 metering systems, and, hence, the financial settlement for the actors along the chain will be more correct. For instance, 1 % accuracy improvement would mean that:
- For the Norwegian full-scale project, the correct settlement implies that values around 200 k€/year are received by the rightful party.
- In DC2030 the correct settlement can imply that 7 M€/year is received by the rightful parties.
For complex CCS chains and systems, the settlements could potentially be larger as there will be multiple parties along the value chains needing custody transfer and thus, depending on accurate fiscal metering.
Learn more about CCS
Join our newsletter to stay updated with all the latest research results and news from NCCS: The Norwegian CCS Research Centre.
Technology providers involved in the development of these innovations are Ansaldo Energia (Case C), and KROHNE and Baker Hughes (Case D). The research is supported by the user industry represented in NCCS: Equinor, Total E&P, Lundin, Vår Energi, Gassco, Norcem, and Oslo. Kommune. The study was conducted by SINTEF, TNO, NGI, NTNU and UiO.
Pingback: Equations of State for CCS: Can We Trust Them? - #SINTEFblog
Pingback: CCS: How Much Does It Cost To Increase Storage Efficiency? - #SINTEFblog