Imagine trying to see and regularly monitor massive volumes of the Earth’s crust without actually going there. It’s a challenge that even the most advanced technology can’t fully overcome. This is exactly what scientists at SINTEF are researching to ensure that when CO2 is stored underground—a crucial step in combating climate change—it is done safely. But that’s not all! The same cutting-edge techniques are being studied to help responsibly extract oil and gas, key pillars of Norway’s economy. At SINTEF’s Formation Physics Lab, we provide crucial research to Norwegian operators, helping them navigate the complexities of the subsurface.
Read the article to find out:
- What is in common between Norwegian architecture and deep geological reservoirs?
- Learn about the most advanced rock sample testing equipment in the world in our laboratories in Trondheim.
- Find out how laboratory tests on tiny rock specimens help us understand the behavior of the subsurface several kilometers deep.
Let’s dive into the science that makes deep underground operations safe and environmentally friendly!
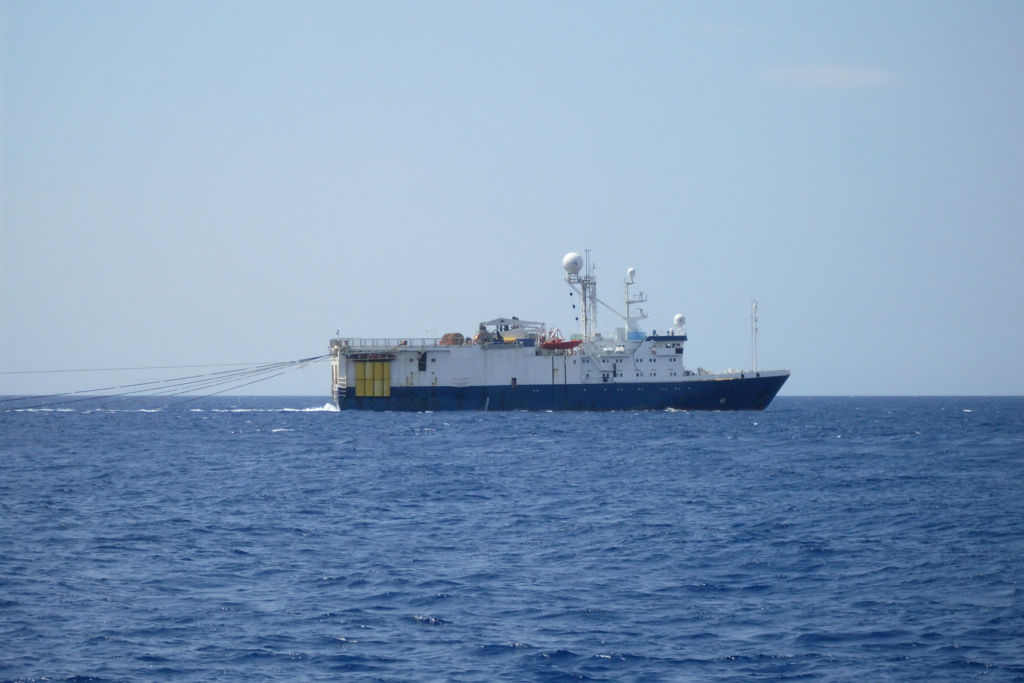
Seismic imaging: a subsurface ultrasound
To illustrate how we can image the subsurface several kilometers deep within the earth, we can compare it to the ultrasound examination a doctor performs on our body. Ultrasound sends small waves (vibrations) and receives reflections from interfaces with different densities (e.g., tissue and bone). The same principle is used in seismic surveys, but on a much larger scale—this way, we get an image of the subsurface showing different types of formations.
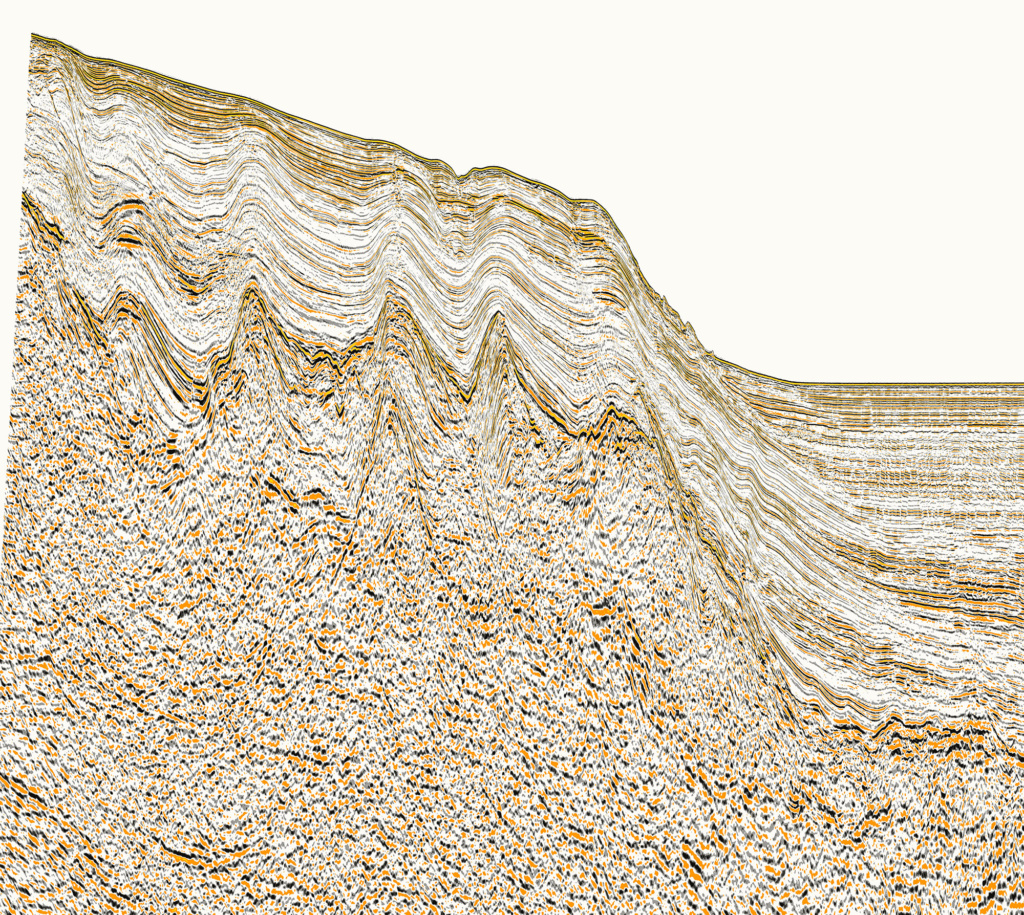
Understanding geological reservoir systems
A geological reservoir that contains oil, gas, or is used for CO2 storage is typically a sandstone or limestone rock with high porosity where fluids are stored. The reservoir is usually surrounded by impermeable rocks that prevent the fluids from escaping (since CO2 or hydrocarbons are lighter than water, they tend to migrate upwards if not blocked by a seal). Impermeable rocks are often shales—the same rock type we commonly see in Norwegian landscapes and use as building material.
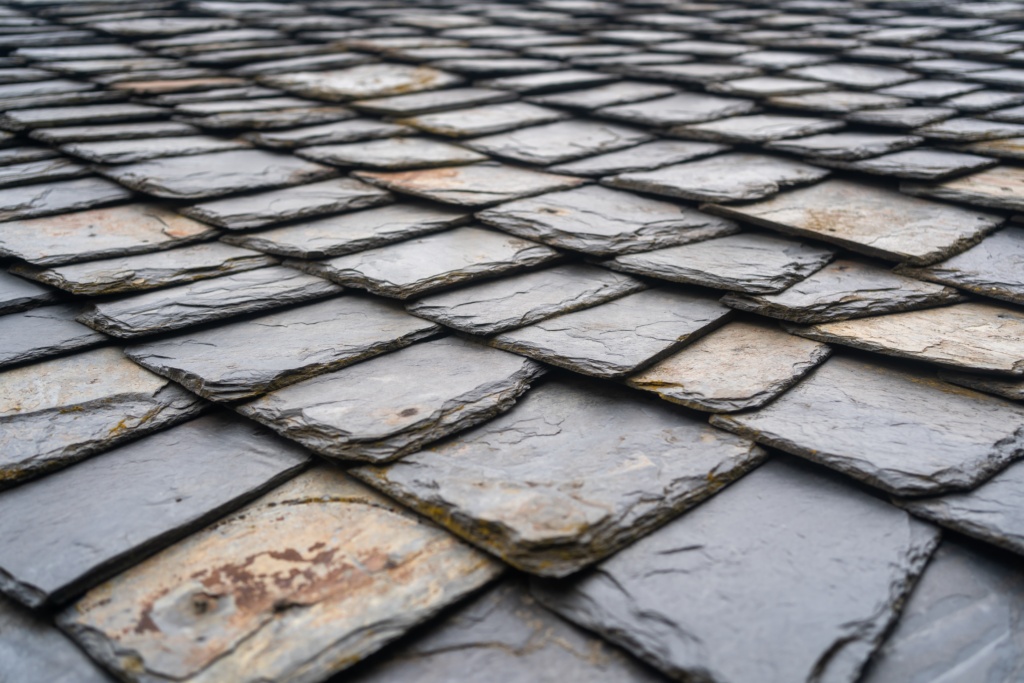
The risks of subsurface pressure changes
So, we have a system: a porous rock reservoir and an impermeable overburden seal. This system remains stable over long geological periods, but when we drill a borehole and start hydrocarbon production or CO2 injection, we significantly change the pressures in both the reservoir and the overburden. These pressure changes lead to deformations that can induce rock failure, resulting in potential borehole and infrastructure collapse, induced seismicity, or even leakage from the reservoir to the earth’s surface.
Monitoring subsurface changes with seismic surveys
To avoid these catastrophic events, we monitor the subsurface with repeated seismic surveys throughout the lifespan of production or injection operations. The main goal is to estimate deformations and pressure changes in the subsurface from variations in seismic wave propagation and evaluate whether these changes exceed safe limits.
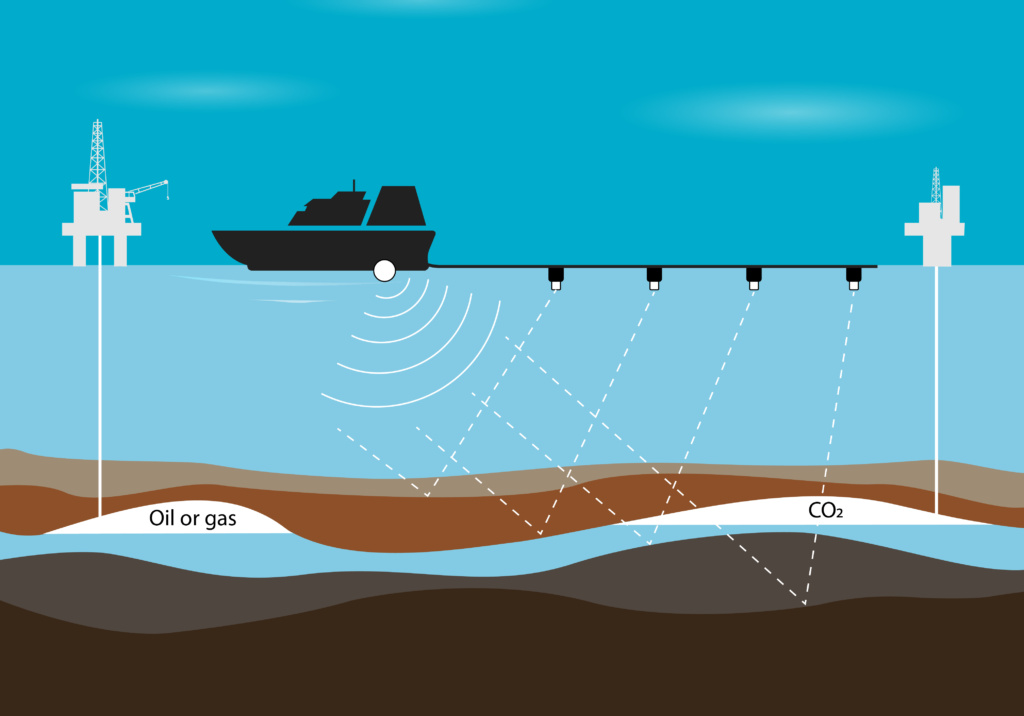
The role of laboratory experiments in subsurface research
A commonly used attribute of seismic surveys is wave velocity—how fast the wave can travel to the reservoir and back to the recording device. Wave velocity changes in rocks that undergo pressure changes and related stress changes and associated deformations. However, we cannot precisely know how velocity changes relate to deformation, which is why scientists study rock samples in the laboratory. Samples are extracted from the subsurface during borehole drilling, and special pressure cells are used to apply equivalent pressure to those found in the subsurface. Then, the pressures are altered according to how they may change in the subsurface during production or injection. During these experiments, wave velocities are recorded and quantified, establishing the relationship between deformations and velocity changes in controlled conditions. These data assist in more accurate seismic data interpretation.
Bridging the gap between lab results and field applications
However, translating lab results into real-world applications remains a significant challenge. Geophysicists observe discrepancies when lab-based models are used to interpret actual field data. At SINTEF Industry, Applied Geoscience Department, we aimed to bridge this gap. Our research focused on how the relation between velocity changes and rock deformations (called R-factor), varies depending on the frequency of propagating waves. This effect is caused by the nature of sound waves – at different frequencies they can travel at different speeds in different media, therefore also change their speed if medium is changed or deformed.
We found indications that the R-factor can be frequency-dependent: lab tests that commonly use high-frequency ultrasonic waves tend to underestimate the velocity sensitivity observed in field conditions, where lower-frequency seismic waves are prevalent.
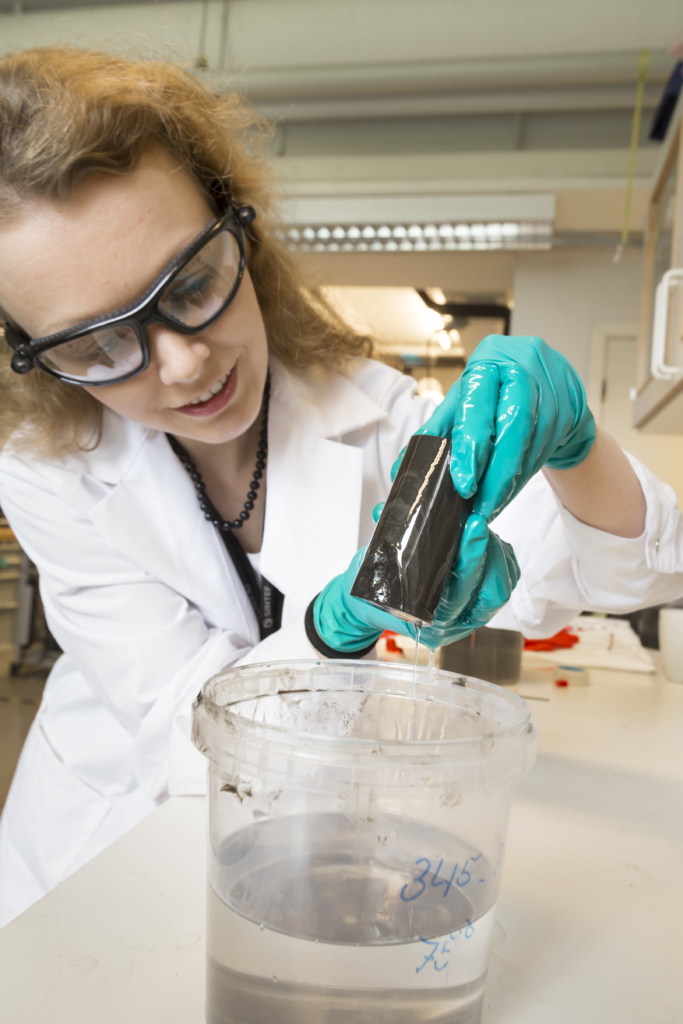
State-of-the-art testing at SINTEF’s formation physics lab
To address this, we utilized a unique low-frequency cell at SINTEF’s Formation Physics Lab, measuring both seismic and ultrasonic velocities in overburden shale samples. This cell is one of the few of its kind in the world and performs state-of-the-art rock characterization.
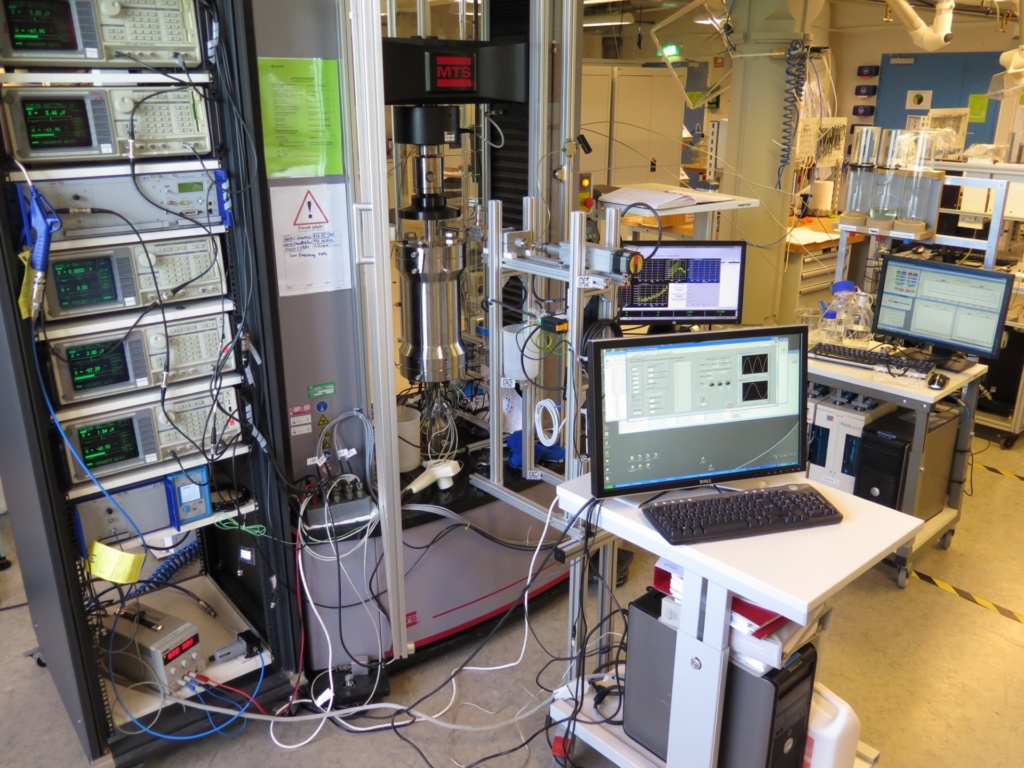
The results were striking: the seismic (low-frequency) measurements showed 2-4 times greater effect of deformations on wave velocities compared to the ultrasonic (high-frequency) measurements.
Importantly, our low-frequency method showed good alignment with field observations reported in the literature, unlike the ultrasonic results, offering a promising path to more accurate connection between seismic survey observations and subsurface deformation and pressure changes.
Moreover, the inconsistency between ultrasonic and seismic frequency results highlights the need for specialized equipment capable of operating at the frequencies that match actual field conditions.
Developing models for accurate predictions
Shales, due to their highly laminated nature, exhibit different properties depending on the direction of wave propagation. Therefore we developed a model that incorporates changes in seismic properties depending on the direction. This model allowed us to estimate model parameters from laboratory tests and accurately predict how seismic velocity changes with deformations induced by changing pressures in the subsurface.
Towards sustainable and responsible subsurface operations
The work we have done not only helps to resolve the disconnect between lab tests and field observations but also underscores the necessity of adapting our experimental approaches to match field conditions as closely as possible. The higher strain sensitivities observed with seismic frequencies suggest that the subsurface is much more responsive to pressure changes than previously thought, especially when inferred from high-frequency ultrasonic tests.
This is a step towards more reliable subsurface monitoring techniques that can help us safely manage and utilize underground resources—whether for CO2 storage, traditional oil and gas production, or even future applications yet to be imagined.
Read our scientific article for more details:
S. Lozovyi, M. Duda, A. Bauer, R. M. Holt, Stress path dependence of time-lapse seismic effects in shales: experimental comparison at seismic and ultrasonic frequencies, Geophysical Journal International, Volume 240, Issue 1, January 2025, Pages 446–461, https://doi.org/10.1093/gji/ggae290
Comments
No comments yet. Be the first to comment!