While we focus on offshore storage under the seabed, many of these principles also apply to underground storage onshore. The National Energy Technology Laboratory (NETL) recently published a technical report (PDF) on safe geologic storage onshore which can be read in conjunction with this post.
What CCS is – and why it matters
Carbon Capture, Transport & Storage (CCS) is a series of technologies and processes that aim to significantly cut carbon dioxide (CO2) emissions. This is achieved by capturing and concentrating waste CO2 from industrial processes instead of releasing it into the air, then transporting and injecting it deep underground. In Norway, this is done beneath the seabed into saline aquifers (porous geological formations containing water) and sealed reservoirs that once held fossil fuels.
While renewable energy and energy efficiency measures have a major part to play in combating climate change, the International Energy Agency (IEA) estimates that energy demand could increase by as much as 45% by 2030. Decarbonizing the non-renewable portion of that energy generation is a must as we move through the green transition. CCS is also the only technology that can decarbonise critical industrial sectors, such as cement and metal production and waste incineration.
The Intergovernmental Panel on Climate Change (IPCC) found that to meet the challenging targets of the Paris Agreement, global CO2 emissions must be reduced by 50-85 % by 2050. IEA findings say that to meet these targets, 14% of the total emissions reduction by 2060 must come from CCS.
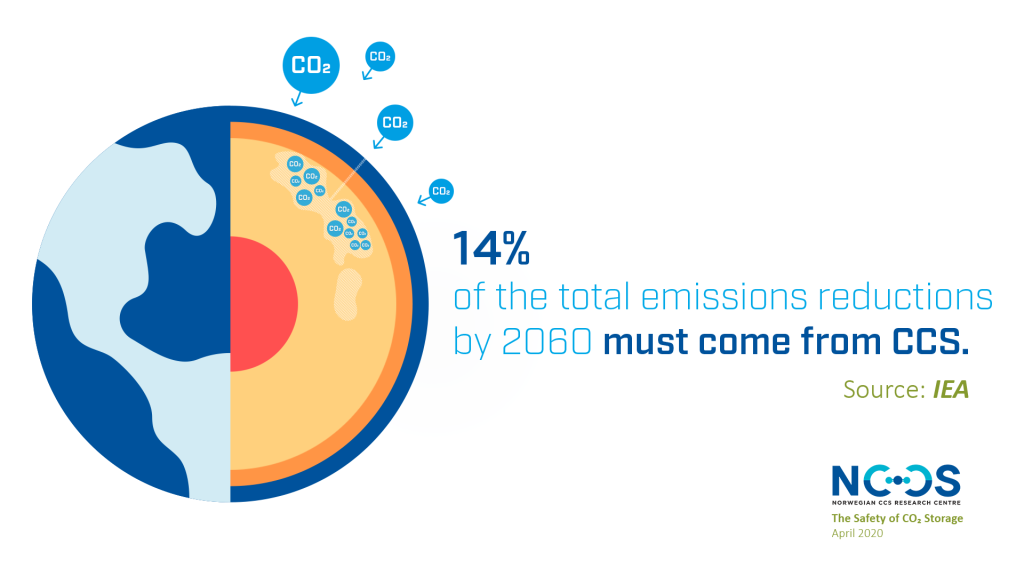
Safe CO2 storage around the world
With vast storage opportunities available around the world combined with decades of relevant industrial experience, CCS can provide a major part of the answer to the climate challenge.
Ongoing industry projects have proven the technology. Several new operational and research projects now underway will improve the technology and processes. For example, the Norwegian CCS Research Centre (NCCS) is working to fast-track CCS deployment by addressing the major barriers identified within the industry demonstration projects. NCCS will run for eight years and is funded by its industrial partners and the Research Council of Norway.
Learn more about CCS
Join our newsletter to stay updated with all the latest research results and news from NCCS: The Norwegian CCS Research Centre.
The key issues of safety and risk of a full-scale CCS implementation in Norway fall into three broad categories, as follows:
1. CCS is proven technology
CCS moved from theory to practical implementation long ago. More than 40 sites around the world have been or are presently involved in safely injecting CO2 into underground storage sites.
Since 1996, the operations run by Equinor and their partners at the Sleipner field in the North Sea has injected CO2 into the Utsira formation. Since then, around one million tonnes of CO2 has been stored in Sleipner every year. Storage has also taken place at the Snøhvit field since 2008.
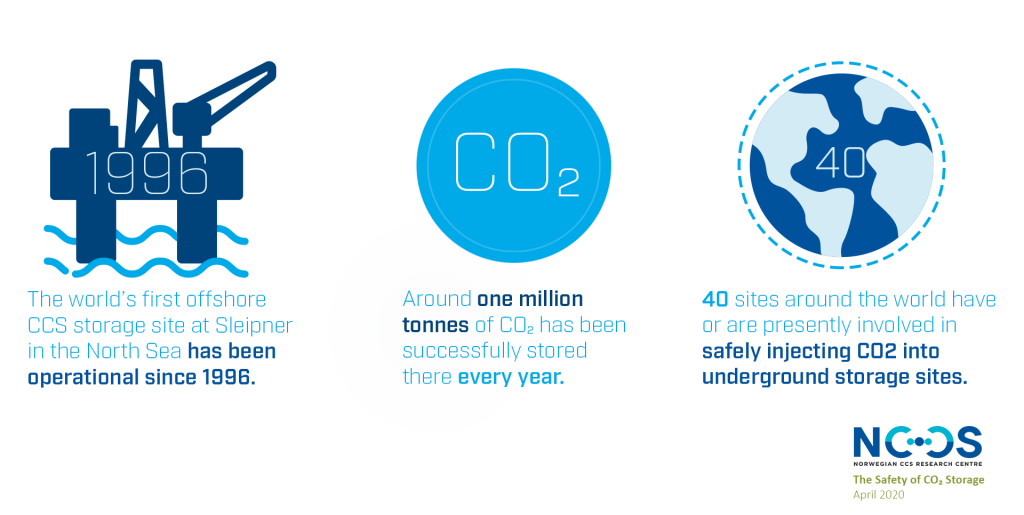
Following a feasibility study from the Norwegian government, a project was launched to develop a full-scale CCS value chain in Norway by 2024.
As part of this initiative, Equinor, Total and Shell are working together on the Northern Lights project that could enable up to 1.5 million tonnes of CO2 storage capacity per year in its first phase. Further expansions, currently limited by the planned pipeline size, could take the available capacity up to 5 million tonnes per year.
The accumulated experience in Norway and around the world has proven that there are no technical barriers preventing the implementation of CO2 storage at scale. Research within NCCS and elsewhere continues to tackle questions related to technical optimisation, overcoming legal barriers, reducing cost, and scaling up operations.
There is no shortage of space, so CO2 storage sites can be carefully chosen
The IEA estimates that more than 100 billion tonnes’ worth of storage capacity is needed to meet the 14% contribution target. A potential capacity of many times more has been identified worldwide.
In Norway alone, theoretical estimates show that 70 billion tonnes of storage is available in saline aquifers and former oil and gas reservoirs, although not all of that is technically or economically feasible to use. If 10% of the storage space were to be utilised, it could provide storage for roughly 40 years of emissions from the European cement industry.
Ongoing NCCS research into reservoirs will reduce the uncertainty on the suitability of many of these sites, providing a more accurate capacity estimate in the years to come.
The abundant capacity worldwide means that we can characterise and select storage sites based on research results and long-established industrial practices, among others. However, this work takes time and needs to be started early in the process.
The storage sites at Sleipner and Snøhvit, and the candidate sites for the Northern Lights project, are all sealed with several hundred metres of cap rock. In some cases, faults in the cap rock for a potential storage site may limit the capacity. New fault seal models being developed in NCCS for site qualification can reduce uncertainty and therefore increase the available storage capacity.
2. CO2 storage is a well-understood, low-risk process
We have many decades of dealing with CO2 in gas or liquid form, and in atmospheric or pressurised environments. Many industries use it for cooling and the production of chemicals and metals, among other uses. The safe handling of CO2 is therefore well-understood.
CO2 is unlikely to escape
The minimum depth for a CO2 storage site is 700 meters below the seabed so that the temperatures and pressures are high enough to keep the CO2 in the liquid or supercritical state. However many projects, including the Northern Lights Project, are as deep as 3,000 metres below the seabed. For well-selected storage sites, there will be several different rock layers (typically shale layers) that provide an impermeable barrier between the CO2 storage reservoir and the seabed.
There are four natural mechanisms that play a role in keeping injected CO2 safely in place:
- Structural trapping: Above the porous rock, impermeable layers of cap rock provide no escape routes
- Residual trapping: Porous rock in the storage site acts like a sponge, trapping droplets of CO2
- Solubility trapping: CO2 dissolves into salt water or brine already present in the porous rock
- Mineral trapping: Over time, dissolved CO2 can react with minerals and bond to rock
These processes are well understood, helping us to characterise and select only the most suitable storage sites at appropriate depths.
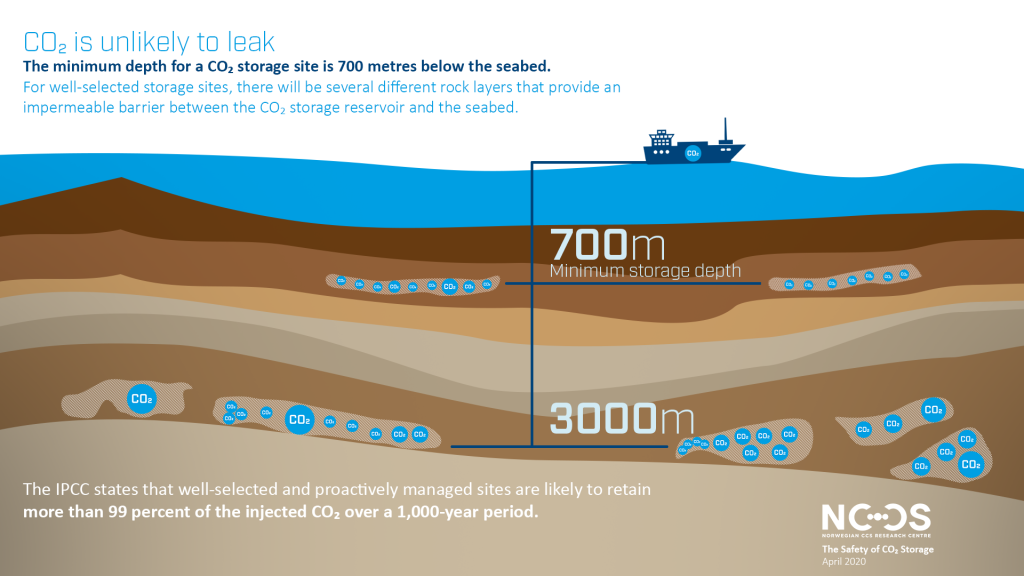
The IPCC1 states that well-selected and proactively managed sites are likely to retain more than 99 percent of the injected CO2 over a 1,000-year period. Since 1996, many millions of tonnes of CO2 have been injected and stored, with no evidence of escaping.
In the unlikely event of a leak, research published in Nature Climate Change2 shows that the impact of any potential CO2 escaping from underground reservoirs is unlikely to cause long-term harm to human life or the environment. Tests showed the biological impact and footprint of a small leak analogue is confined to a few tens of metres.
CO2 storage sites are closely modelled and monitored
In addition to natural mechanisms keepingCO2 safely trapped, storage sites are closely monitored for any evidence of CO2 migrating towards the surface. There are a range of mechanisms to control and prevent an identified risk from becoming a leak, which are based on decades of operational experience.
In Norway, legal requirements are in place for the monitoring of CO2 storage sites that go beyond what is required for the established oil and gas industry. Operators must demonstrate conformance to regulations and assurance of containment. At the Sleipner site, there is a requirement to monitor the seabed to detect any CO2 leakage.
CO2 storage operators make seismic data and other measurements available to researchers worldwide, including in NCCS. This allows the testing, iteration and improvement of imaging methods and data models for future projects. NCCS researchers have been able to work together with industry experts analysing monitoring data from both Sleipner and in the future, the Northern Lights project.
Such monitoring data also proved that the biogenic gas detected to be leaking from the Hugin fracture in 2012 had no relation to the storage operations at Sleipner. At the Sleipner site, independent researchers analysed data using seismic-reflection surveys of the deep subsurface before CO2 injection and then at two-year intervals. They found excellent performance of the storage site, with no evidence of any CO2 leakage. Analysis of the Hugin fracture site, located 25km northeast of Sleipner, was presented in a technical paper3 at Near Surface Geoscience 2014 – 20th European Meeting of Environmental and Engineering Geophysics.
Monitoring data were also used effectively to de-risk storage at Snøhvit, allowing the team to modify the injection to access a better reservoir when pressure build up was detected early.
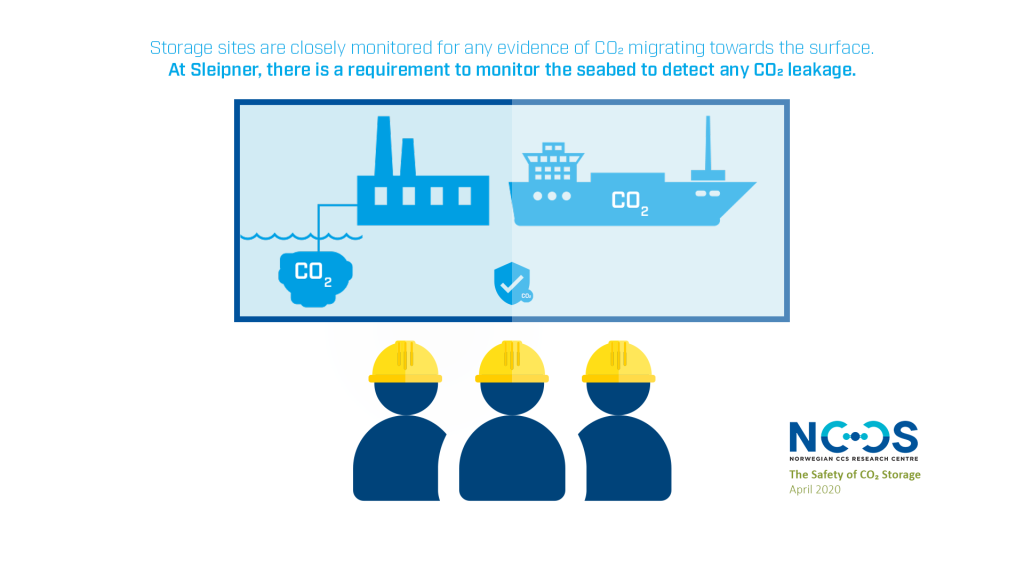
The behaviour of CO2 in underground storage
We also understand the characteristics of stored CO2 from the naturally occurring CO2 that has been trapped underground for many thousands of years.
NCCS researchers are also looking at how to increase the amount of CO2 that can be stored safely in certain formations, which will feed into strategic decision-making around site selection. One study presented at the 10th Trondheim CCS Conference in 2019 shows how chemicals developed for mobility control could cause CO2 to displace water more easily, optimising the storage capacity of an aquifer.
Building on methodology from natural gas distribution networks, a new risk calculation tool helps with strategic storage optimisation and a long-term risk analysis, removing some of the financial uncertainty.
Solid understanding of well integrity
The wells themselves remain the most likely cause of potential leakage because of the artificial (man-made) routes from reservoir to the seabed. Injection of CO2 will cool the rock close to the well. Research is ongoing into the effects of the resulting thermal stresses in proximity to the well. Much NCCS research is focused on how to avoid seepage near the well by ensuring there are multiple barriers between the stored CO2 and the surface.
Research into the quality of cement used in oil wells has been done for more than 20 years. NCCS researchers have studied the characteristics of cement with exposure to CO2, in particular in old, abandoned wells that may be located close to some potential storage sites. Results show that the degradation of cement, if it were to happen at all, would be just a few millimetres over the course of a thousand years.
Learn more about CCS
Join our newsletter to stay updated with all the latest research results and news from NCCS: The Norwegian CCS Research Centre.
NCCS has made recommendations for both optimal design and selection of materials for new wells in addition to evaluation of legacy wells. A spin-off project from NCCS will continue to look at integrity monitoring of old wells together with industry partners.
How CO2 behaves during transport
We understand a lot about its behaviour in pipelines from decades of natural gas industry experience. Today, CO₂ is transported through a 150km-long seabed pipeline from the Snøhvit field to the Norwegian mainland. In North America, there are thousands of kilometres of CO2 pipeline installed and operational. Results from NCCS research into pipeline corrosion have been used by Equinor in the Northern Lights project.
As an alternative to pipelines, shipping of CO2 (at roughly 15 bar) is a mature technology. Norwegian company Yara International has a fleet of tanker vessels dedicated to transporting CO2. Each one can carry up to 1,800 tonnes of liquid CO2 safely and cost-efficiently. For the Northern Lights project, the 15-bar pressure shipping option has been selected. For future solutions, NCCS has suggested that lower shipping pressures can potentially be a safe option and can decrease the cost of CO2 transport significantly.
3. The seismic risks of CO2 injection and storage
Many human activities carry a risk of inducing minor seismic activity, including oil and gas operations and geothermal energy production. However, the scale of seismic activity required to cause an earthquake that can be felt by humans (generally above magnitude 4) is generally only possible through natural forces.
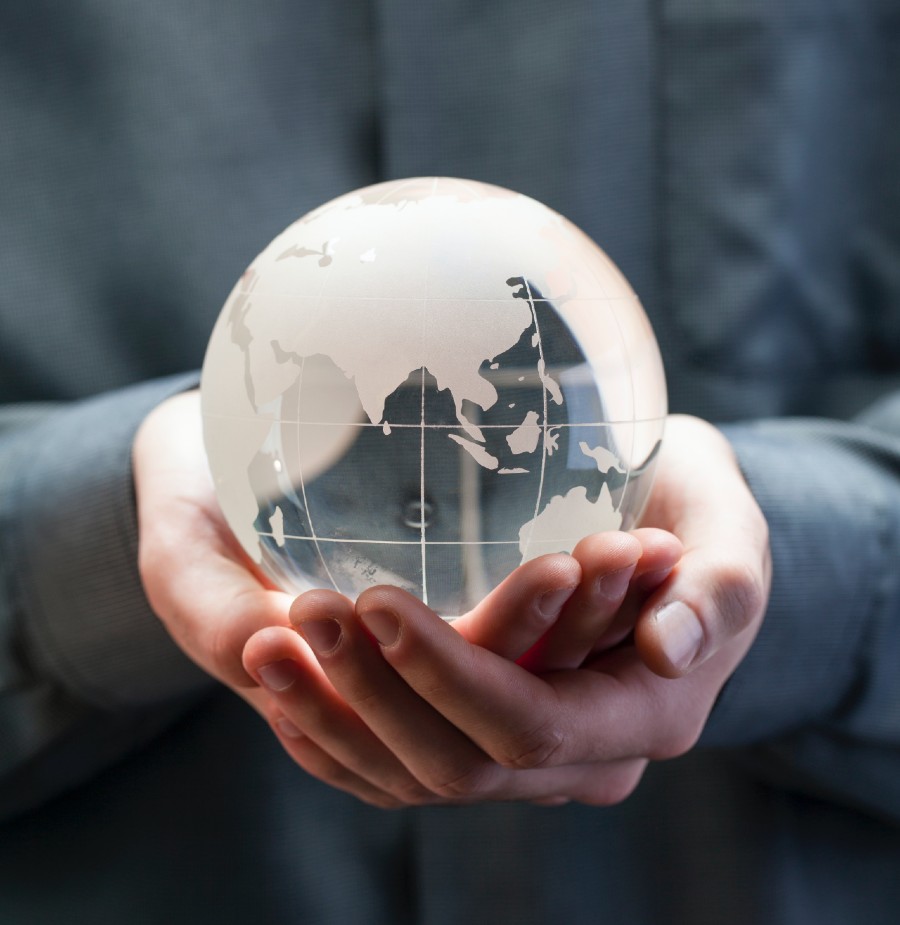
In the event of seismic activities, the risk to CO2 storage is low. North Sea oil and gas reservoirs regularly experience minor earthquakes, with no detected leaks of oil and gas caused by the seismic activity. Recently a magnitude 6 earthquake occurred near a pilot CO2 storage site in Japan. The earthquake was proven unrelated to the injection process and the storage site was so secure that no leak of CO2 was detected.
Managing fault activation risk through pressure limits
The topic of fault activation (induced seismicity) has been intensely studied in recent years in relation to hydraulic fracturing. NCCS research is addressing the risks of fault reactivation in the context of CCS. Models are being developed to assess the impact of different levels of injection pressure in order to define a safe pressure limit. In the United States, injection- induced seismicity and micro-earthquakes have been studied extensively in the Illinois- Basin Decatur project. Results have shown that these events occur often too deep to affect the integrity of the caprock or allow CO2 to escape.
Our experience from CCS demonstration projects in Norway show that micro-seismic events are both low in frequency and magnitude. As micro-seismic activity could be an early indicator of bigger seismic events, other research projects related to this topic are ongoing within SINTEF, the Norwegian Geotechnical Institute and the Norwegian Seismic Array, NORSAR.
Conclusion: CO2 storage is a safe solution for the climate challenge
With deep understanding of the gas in all its forms and decades of relevant industrial experience, CO2 storage in Norway is a safe option for the decarbonisation of industry across Europe. With rigorous procedures for the selection, operation and monitoring of selected storage sites, the safety bar for Norwegian CO2 storage sites is extremely high.
The storage capacity and expertise available in Norway means the country is well-positioned to be a CO2 storage provider for much of Europe. NCCS research is building on that basis to provide additional insights to create a safer, cheaper operation at scale.
[blue_box]
About NCCS
The Norwegian CCS Research Centre is an FME (a centre of excellence under the Research centre for environmentally- friendly energy research) will enable fast-track CCS deployment through industry-driven science-based innovation, addressing the major barriers identified within demonstration and industry projects, aiming to become a world-leading CCS centre.
NCCS aims to support achieving CO2 storage in the North Sea, contributing to the Norwegian government’s ambition to realize a full-scale CCS chain by 2024. In addition to research activities on CO2 storage and transport, NCCS addresses CO2 capture technologies and the CCS value chain. It is a long-term, stable research collaboration driven by the needs of Norwegian industry, while building strategic partnerships in areas of common international interest.
Co-financed by the Research Council of Norway, industry, and research partners, NCCS runs until 2024.
[/blue_box]
Authors
Amy Brunsvold, Mona Mølnvik, Grethe Tangen, Inna Kim, Philip Ringrose, Elin Skurtveit, Pierre Cerasi, Peder Eliasson, Alv-Arne Grimstad, David Nikel
References
- IPCC Special Report: Carbon Dioxide Capture and Storage Summary for Policymakers.
- Blackford, J., Stahl, H., Bull, J. M., Bergès, B. J., Cevatoglu, M., Lichtschlag, A., … & Naylor, M. (2014). Detection and impacts of leakage from sub-seafloor deep geological carbon dioxide storage. Nature climate change, 4(11), 1011-1016.
- Near Surface Geoscience 2014 – 20th European Meeting of Environmental and Engineering Geophysics.
- Safe Geologic Storage of Captured Carbon Dioxide: Two Decades of DOE’s Carbon Storage R&D Program in Review, National Energy and Technology Laboratory (NETL) report, April 13, 2020.
Learn more about CCS
Join our newsletter to stay updated with all the latest research results and news from NCCS: The Norwegian CCS Research Centre.
Comments
Just for info we provide cost efficient ultra high temperature Pulsed Neutron Capture Logging and Monitoring Co2 storage fields and geotermal wells.
Jeg håper Mølnvik og Tangen skriftlig vil kommentere nedenstående bemerkning til CO2 og klimaendringer, relatert CCS og kostnader for samfunnet. Et samfunn der mange barn lider pga. mangel av økonomiske midler.
Bemerkning til CO2, klimaendringer og klimapolitikk:
xxx
Antall molekyler i 1 m3 luft ved bakkenivå er: A= 2.7 x 10 (25) (potens)
Antall molekyler CO2 (400 ppm) av alle molekyler: B= 2.7 x 10 (25) x (400 x 10 (-6) ) = 10,8 x 10 (21)
Antall molekyler CO2 fra fossilt brensel (4%) av alle CO2 molekyler er:
C= 10,8 x 10 (21) x 4 x 10 (-2) = 43,2 x 10 (19)
Hvert CO2 molekyl kan absorbere: D= 1,33 x 10 (-20) Joule (wattsekund energi) (1 Joule = 1 J)
Energi absorbert av CO2 molekyler fra fossilt brensel i 1 m3 luft: E= D x C = 57,46 x 10 (-1) = 5,75J
Ergo: CO2 molekyler fra fossilt brensel i 1 m3 luft absorberer: 5,75J
Fordampningsvarmen for vann ved +15 oC er: F= 2470 J / gram
Vekt av vann som fordamper ved +15 oC og relativ fuktighet i luft 60% gir vekt av fordampet vann lik
G = 7,6 gram.
Energi som vanndamp tar med seg opp i atmosfæren, og som frigis ved kondensering
(skyer, nedbør) er: H = F x G= 2470 J /gram x 7,6 gram = 18 772 J
Av dette finner en at den relative mengde energi CO2 fra fossilt brensel, i forhold til energi fra fordampet vann, bringer inn i 1 m3 av atmosfæren, er:
I = E: H = 5,75: 18 722 = 0, 000307
Ergo: energien som CO2 fra fossilt brensel fører inn i atmosfæren er bare 0,3 promille av det fordampet vann fører inn under de gitte betingelser med relativ fuktighet 60% og atmosfæretemperatur lik +15 oC. Tilført mengde energi øker med stigende lufttemperatur og med økt relativ fuktighet.
Dette viser at dersom fordampning av vann endres med en promille så vil dette overskygge den energimengde som en fordobling (til 800 ppm) av CO2 fører inn i atmosfæren. Energi er drivkraft til endring av vær og klima.
Vennlig hilsen
Kjell B. Mortensen
(tidligere medarbeider ved EFI/ SINTEF)
PS: Jeg venter en kommentar! DS