Modeling and predictions are used to make political goals for energy efficiency in buildings. To ensure that the goals are realistic, we should use open data. Buildings stand for more than a third of the total energy use in Norway and more than 50% of electricity use. This means that energy efficiency measures in buildings will have a great impact.
The current political goal in Norway is to save 10 TWh by 2030, only from buildings. The goal is based on models whose assumptions and datasets are often not fully transparent. That makes it hard to verify or challenge the calculations or specify which measures will have the biggest impact and at what timing.
Listen to our podcast on this subject
A dynamic building stock model
SINTEF and NTNU have worked on a model based on open data for several years, beginning with the Residential building sector (see Sartori et al., 2016 for a reference) and then extending the model to include also the Service building sector (see Sandberg et al., 2021 for a reference). The model is now called RE-BUILDS and is continuously under development; the figure below shows a schematic layout of the entire modes. This dynamic model provides a deep understanding of the dynamics driving the development of the building stock over a long period. The driving force in the model is a population’s need for housing and working/service space, and the necessary input is retrievable from national statistics on population, often dating back to around 1800, and its prognoses up to 2050 or beyond.
To verify the model, we have started from 2020 and then “backcasted” (the opposite of forecasting) the model results for 2015 and 2010, observing a good match with statistics on energy use in all years. With this method, we have observed that also the dynamics appears calibrated, not only the values in a single point in time.
The model allows detailed analysis of the building stock’s size and composition, and of the construction, renovation, and demolition activities: how they developed over time and what the future projections look like. The model’s description is fully transparent, and the core algorithm is described and presented in the references given above, and in a number of other peer-reviewed papers and PhD theses (Sartori, 2008; Bergsdal, 2009; Sandberg, 2017).
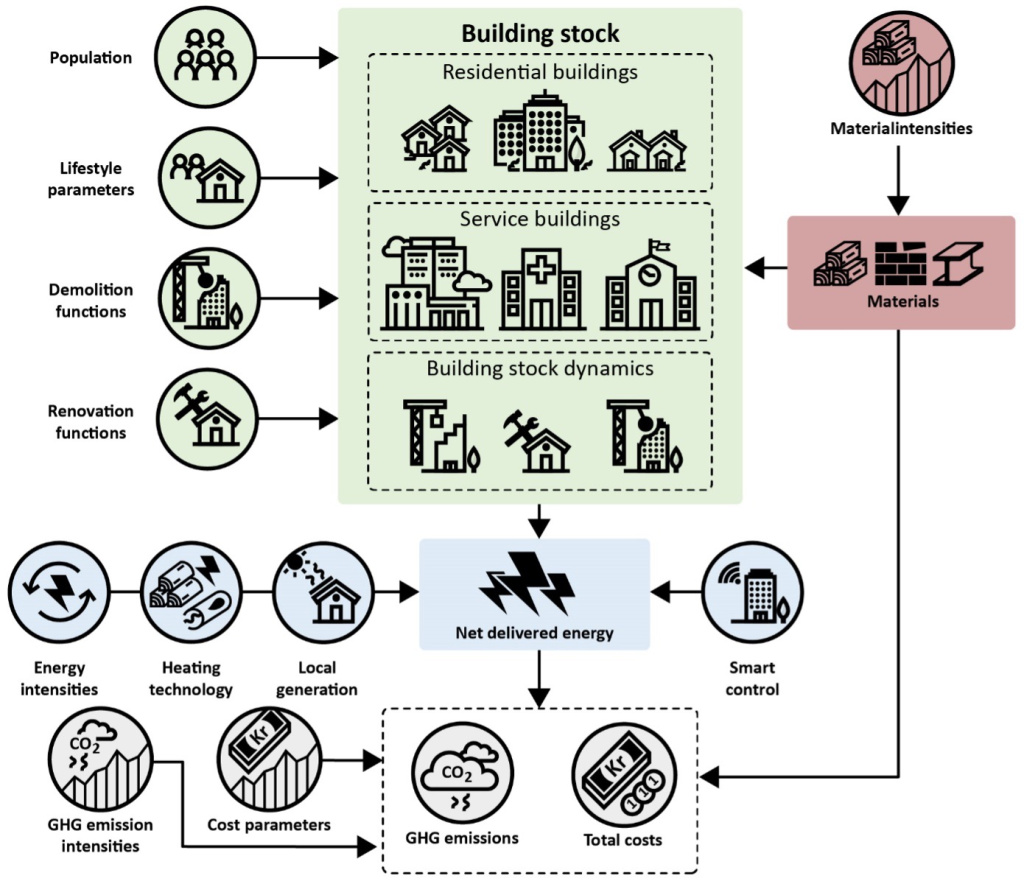
The model is of general validity and relies on input data from population statistics available in principle in any country. The model thus provides a solid basis for studying the related impacts on energy demand, GHG emissions, materials demand, and waste flows.
Technical parameters such as the buildings’ lifetime and the renovation cycles are expressed by probability functions. Outputs of the model are the flows of construction, demolition, and renovation; analysis of the renovation activity is given particular attention.
The model is tested on European countries
The model (on the residential sector only) has also been used to simulate the development of dwelling stocks in 11 European countries between 1900 and 2050. Despite differences in data collection and reporting, the model showed similar patterns in all countries regarding future trends for construction, demolition, and renovation activities (Sandberg et al., 2016).
The model estimates future renovation activity due to the ageing stock’s need for maintenance. The simulations show only moderate and slow future increases in the renovation rates across all 11 countries, remaining in today’s range of 0.6–1.6% (When expressed as % of the stock size in each year. If we keep the year 2015 as the fix reference, then the renovation rate by 2050 will be in the range 1.4–2.0% of it.). That is significantly lower than the assumption of a 2.5–3.0% renovation rate adopted, for the entire modeling period, in many decarbonization scenarios.
The calculations show that 78% of all dwellings could benefit from energy efficiency measures by 2050, either as they are constructed (31%) or undergo deep renovation (47%). However, as no more than one deep renovation cycle is likely in this timeframe (for each and every single building), it is crucial to install the most energy-efficient measures available at these opportunities.
Energy efficiency potential in Norway
In a recent article (Sandberg et al., 2022), we have used the model to estimate the energy saving potential in the Norwegian building stock in the period 2015-2050. We considered 3 scenarios. The first is the baseline, where the recent and present trends continue. In this scenario, only 20% of the renovations include energy efficiency measures (one building out of every five renovated, in average). This leads to an increase in energy demand of 3 TWh by 2030 and beyond.
The second scenario shows the potential for energy efficiency by assuming that all renovation will be carried out, starting from today, with substantial improvement of the building envelope, bringing older buildings to approximately the same standard as new ones. The data for the energy demand before and after such renovations come from yet another tool, called PROFet (Andersen et al., 2021) and itself under continuous development within the research centre on Zero Emission Neighbourhoods in Smart Cities (https://fmezen.no/). This is a statistical extrapolation from a database of measured energy consumption from many buildings, covering a total floor area of ca. 2.5 million m2 (square meters), with hourly resolution, over several years of observation. The result of the second scenario is that energy demand by 2030 can be leveled off, i.e. remain at the same level as today, and even decrease by 4 TWh by 2050, while the building stock keeps on growing due to the expected population growth and urbanization process.
The third and last scenario adds the introduction of water-based heat pump systems (with electric top-heater) in all new and renovated buildings. These are the most efficient heat pumps, but they work best in well-insulated buildings and require a waterborne heating system (radiators or floor heating); i.e. not the standard in Norway, where most building use direct electric heating with electric panels. Since it is an intrusive measure to introduce a waterborne heating system in existing buildings, we have limited it to those that will undergo renovation. Installation of simpler, and less efficient, air-to-air heat pumps in small houses, as a complement to existing electric panels is also considered. The results in this scenario indicate a potential decrease of 10 TWh by 2030, cumulating to 20 TWh by 2050.
Incentives and regulation are needed to increase efforts
It is common to assume that the present renovation rate is around 1%, and this is confirmed by the results from our model. But this assumption is widely followed by advocating that the renovation rate should double, or even triple, in the span of a few years.
Our model shows that such hypotheses are unlikely. The aging building stock will need more and more renovation, but this “naturally occurring” renovation rate will not be more than ca. 1.5% by 2050.
Today, in average, only 1 in 5 renovations include significant energy efficiency measures, i.e. only 0.2%. To harvest the energy saving potential we should take a big leap forward; and that requires strong incentives and the removal of barriers such as financial, technical, and regulatory. The benefits, on the other hand, are large because more efficient buildings free up energy resources and grid capacity that can be used to cover other needs of the society, like the electrification of transport.
Alas, it will take time to build it up. The building stock can change significantly – as it always has done, after all – but cannot do it faster than such. This is one aspect that our model seems to capture. The lesson that can be learnt is that political goals and policy making should also be devised with a long-term perspective in mind. Striving to harvest gradual changes would be a wiser and more realistic approach than deluding ourselves with short term frenzies.
Comments
No comments yet. Be the first to comment!