According to the International Energy Agency (IEA), CO2 Capture and Storage (CCS) is the third most important measure to limit the global warming by 2°C. An important part of the CCS chain is the transport of CO2 from points of capture (e.g. power plants) to points of injection and storage. CO2 transport will likely happen by pipeline and/or shipping. In the BIGCCS International CCS Research Centre, research has been performed on how to make CO2 pipeline transport both safe and cost-efficient.
Under pressure
In order to transport sufficient amounts of CO2 in reasonably large pipelines, the CO2 must be compressed to a liquid state, at pressures around 100 atmospheres. One of the safety-aspects in high-pressure pipeline transport is the danger of an incident called running ductile fracture (RDF). In such an event, some small initial fracture in the pipeline can start running along the pipeline in both directions. In the worst case, this may potentially split hundreds of meters of pipeline, causing large and dangerous leaks.
(…) some small initial fracture in the pipeline can start running along the pipeline in both directions. In the worst case, this may potentially split hundreds of meters of pipeline, causing large and dangerous leaks.
Thicker steel is expensive
An important design-criterion for pipelines is then to make sure that such a running fracture will stop early (“arrest”), as opposed to run a long distance (“no arrest”), for the given transported fluid. This may be done by e.g. using stronger or thicker steel. However, stronger or thicker steel is expensive, so being overly safe will make the pipelines unnecessarily expensive, and thus make CCS implementation less likely to happen. Because of this, predicting the threshold between arrest and no-arrest is important to enable safe and cost-efficient CO2 transport.
Old methods – incorrect results?
This has been an issue in natural gas transport for a long time, and semi-empirical two-curve methods have been developed to predict the mentioned threshold. However, there is an important difference compared to CO2. Natural gas will expand from high pressures smoothly, while CO2 will experience a liquid-to-gas phase transition. This has great consequences for how the pressure evolves in the event, and thus also for the RDF phenomenon. There are indications that the old methods give incorrect results for CO2 transport, and that something new is needed.
In response to this, SINTEF Energy Research and SINTEF Materials & Chemistry has a collaborative effort in the BIGCCS Centre to develop a more advanced model for predicting RDF. This model aims to describe the phenomenon in much greated detail, using a tight coupling between structural/fracture dynamics and fluid/thermo dynamics.
Results will be presented on TCCS-8
Recent developments within this work will be presented on TCCS-8 on June 17. Below we see videos of two simulations, the first being no-arrest, and the second with arrest.
No arrest
Test #1: Visualization of a simulated running ductile fracture in a CO2 pipeline. In this case, the combination of pipeline and fluid properties causes a running ductile fracture.
With arrest
Test #2: Visualization of a simulated running ductile fracture in a CO2 pipeline. In this case, the combination of pipeline and fluid properties causes the fracture to arrest early
In these two tests, the pipelines are the same. So why does the fracture arrest in Test #2, but not in Test #1? The difference lies in the initial temperature and pressure of the CO2. As shown in the figure below, this leads to different saturation-pressures, which is one of the most important driving forces behind RDF. Surprisingly and counter-intuitively, the test with the highest initial pressure (Test #2) is actually safer with respect to RDF, due to it leading to a lower saturation pressure. This illustrates the crucial importance of thermodynamic predictions when modelling RDF.
Surprisingly and counter-intuitively, the test with the highest initial pressure (Test #2) is actually safer with respect to RDF, due to it leading to a lower saturation pressure.
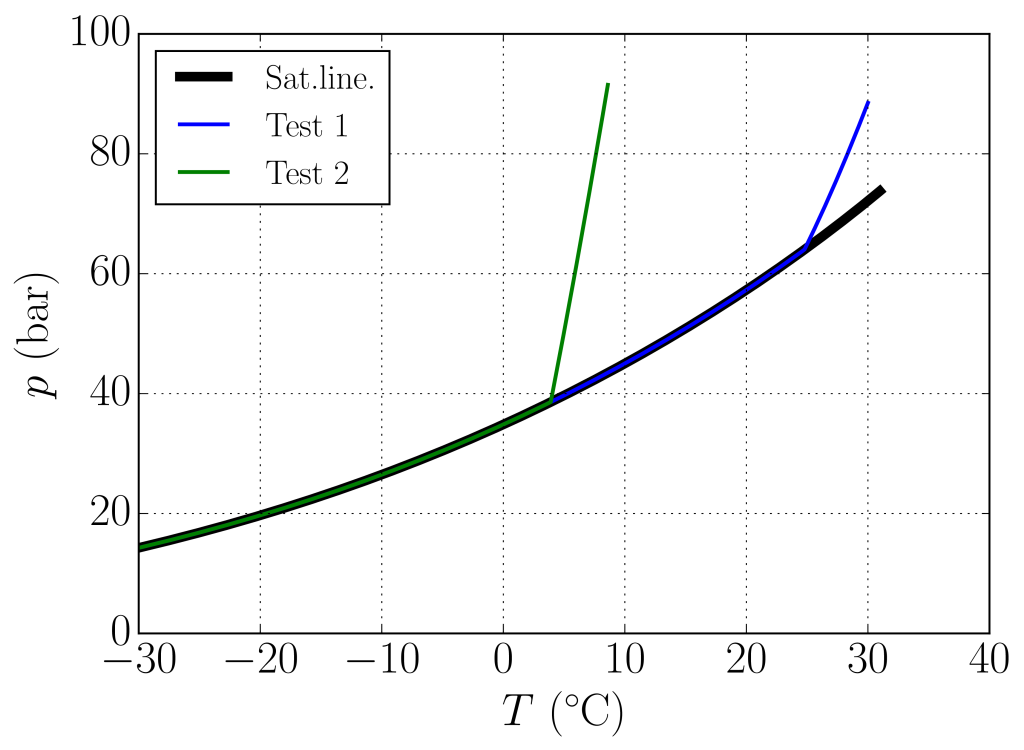
Find out more about our research on CO2 transport in these blogs:
- Models, data and software for safe and efficient CO2 transport
- Why good models are important for safe and efficient CO2 transport
- Flow in CO2 pipelines
This work has been done with support from the BIGCCS Centre, performed under the Norwegian research program Centres for Environment-friendly Energy Research (FME). The authors acknowledge the following partners for their contributions: Gassco, Shell, Statoil, TOTAL, GDF SUEZ and The Research Council of Norway.
Comments
It is mentioned in the article that the latest updates of the work would be presented in TCCS-8. Is this published somewhere where I could have access to it?
The best reference for the work we talk about here is our article in Engineering Structures, http://dx.doi.org/10.1016/j.engstruct.2016.05.012. It can also be downloaded from our web site, https://www.sintef.no/en/publications/publication/1356621/.
I also recommend you check our new work in https://doi.org/10.1016/j.psep.2023.01.054.
Is it possible RDF to propagate as long as kilometers?
In a worst-case scenario it could happen, although in practice, the fracture would likely arrest earlier, due to joints, bends or other features. Also please note that the CO2 pipelines that we are aware of, have an intrinsic high fracture resitance or are additionally equipped with crack arrestors, so that a long running fracture would not occur.
How does steel grade influence the above results? Do you recommend grades x70 and above for CO2 applications for thinner wall thickness? Are there any chemistry/ hardness limitations to the steels to like NACE H2S service?
The results are expected to be sensitive to steel grade, especially to CVN, but there are few data for this. I’m afraid we cannot give general recommendations at this point.