I conduct research into the silicon we use in solar cells. My research will contribute towards getting more energy from sunlight. In this blog you can find out more about how I do it.
I work with silicon – the actual material that solar cells are made of. Silicon captures the sun’s rays and converts light into electricity. We call this a photoelectric effect. Einstein explained this as early as in 1905, and was later awarded the Nobel Prize in Physics for this work (after the Nobel Committee omitted to honour him for his theory of general relativity).
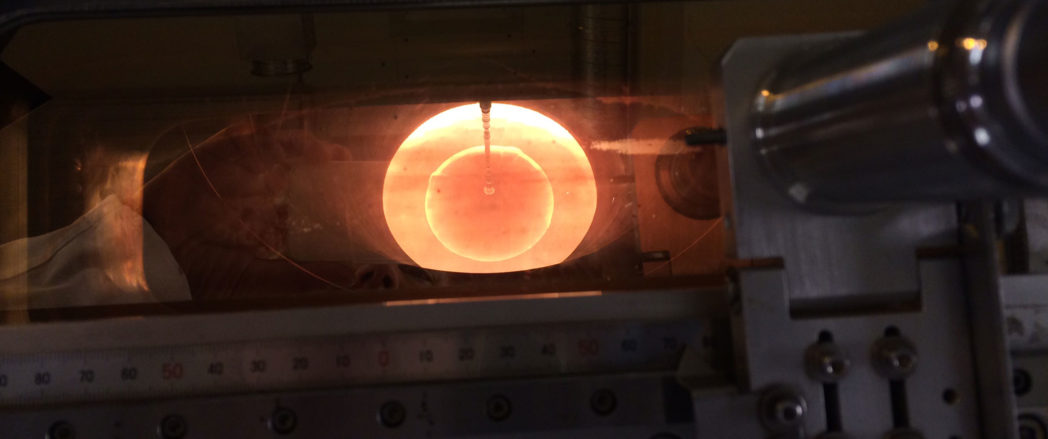
In other words, we’ve known about this effect for a long time and it’s been the subject of research for many years. We deal in complicated terms such as quantum mechanics, photons, electrons, semiconductors, band gaps and crystalline structures.
A silicon crystal is full of defects!
Silicon is one of our planet’s most widespread elements and, in its purest form, binds its atoms together to create an ordered pattern in the form of a crystal with a precisely-defined crystalline structure.
Nature is fantastic and fascinating, but it’s also complicated. Not all silicon atoms necessarily align themselves neatly like pearls on a string. Even though every energy computation we know of indicates that it would be smart (or as we like to say – energetically favourable) for silicon atoms to line up according to a pre-ordained crystalline structure, this isn’t always the case. A silicon crystal is full of defects!
Different parts of the crystal may adopt different orientations, and where these parts meet grain boundaries are formed. Even worse, when the atoms in one crystal fragment align themselves a little differently, the defect propagates to form what we call dislocations.
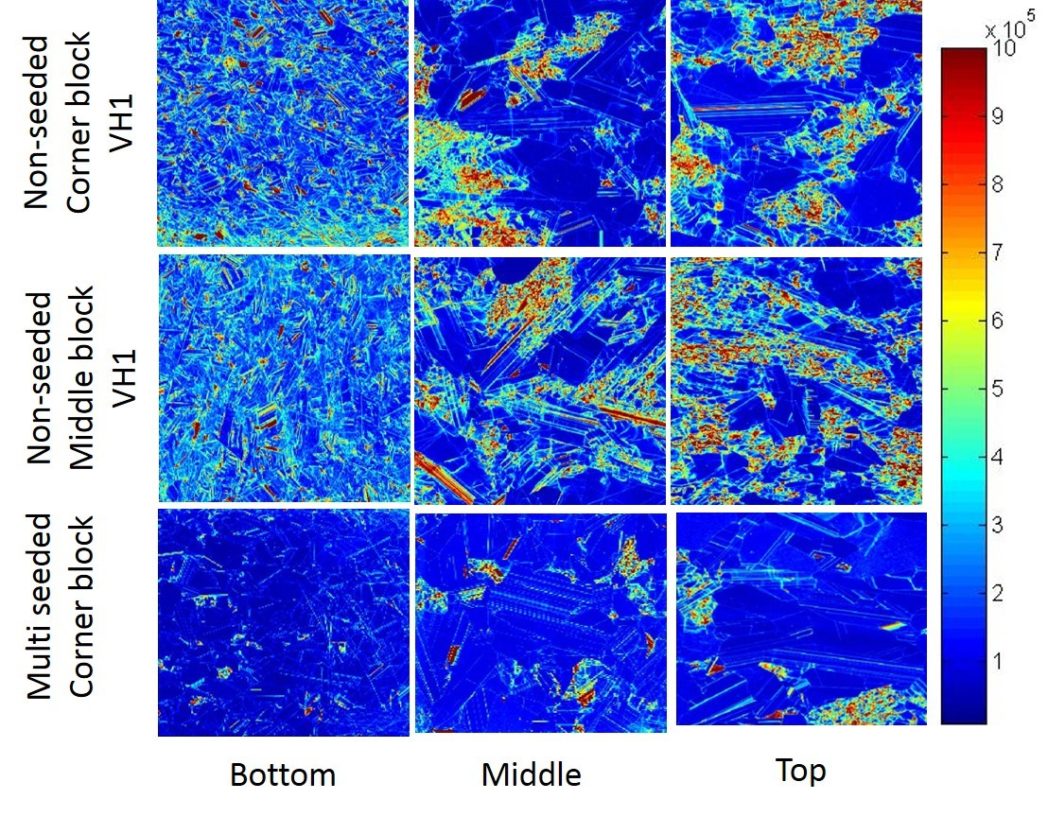
Dislocations – stepping out of line
Dislocations arise from atoms that are not properly aligned in what is otherwise a well-ordered atomic lattice. Just like when a trumpeter in a military band steps out of line or, even worse, if all the cornet players suddenly moved a few steps from the other musicians or if the drummer in a school band got caught up in the ranks.
If this isn’t enough, 100% pure silicon doesn’t even exist – in the same way that no vacuum exists, at least on Earth. True enough, we’re working with what we call the “nine Ns” – that is to say a purity of 99.9999999%.
This is somewhat difficult to achieve because it is very demanding on resources, although both the solar cell and electronics industries produce tons of the stuff each and every day. It costs time, expertise and, not least, energy in order to produce (almost) pure silicon.

A little less pure, but much less expensive
But what neither we nor the industry have succeeded in tackling (yet) is the problem of dislocations. There exists one less pure form of silicon, composed of many crystals, that we call multicrystalline silicon, which is cheaper to manufacture and easier to mass-produce.
Seen in the context of today’s massive growth in the solar cell industry, operating in a market with enormous pressure on prices, multicrystalline silicon represents an attractive alternative, and is in fact the most commonly used.
So, what about these dislocations, or all these musicians who keep stepping out of line? They generate noise, and reduce efficiency when incorporated into solar cells. This means that we obtain less electricity from sunlight than we would have done if we had used a perfect crystal.
Just like knitting
In any case, it is these dislocations that keep me occupied for much of my working day. They are no bigger than atoms but can extend over large distances – up to many metres in length! It’s like dropping a stitch when you’ve almost finished knitting a jumper. Just one little stitch lost and the whole thing tears away and ruins all that work!
And no-one knows for certain how dislocations form, how they behave during manufacture, or what happens to them when they are cooled. There’s no shortage of theories, formulas, models, explanations and opinions. All of them are equally complex, but there is no consensus.
The result is that the industry is in the dark as to what part of the manufacturing process it should optimise. My colleagues and I are working to find explanations that will help towards the survival of the Norwegian silicon industry, obtain more energy from sunlight and, to put it simply, make a small contribution towards saving the planet. Or, as we say here at SINTEF, to develop technology for a better society.
Comments
No comments yet. Be the first to comment!