Recently, I attended the Bioenergidagene (Bioenergy Days) conference and gave a presentation on biofuels. I was asked many questions during the event and in particular about the different kinds of biofuels and their classifications.
The definition of a biofuel is very simple. It’s a fuel that is made of biological raw materials.
The “problem” with biofuels is that the same fuel can be produced from several different raw materials, and a single raw material can be used to make many kinds of fuel of different qualities. It’s a real jungle out there!
- From Gemini.no: Do biofuels contribute to a better environment? Er biodrivstoff et bra miljøtiltak? – in norwegian
There are biofuels and biofuels
Many things in this world are called biofuels.
If you think about wind energy, for instance, things are much simpler. If the wind blows hard enough and you’ve installed a turbine, you can generate electricity. Of course, there are many different approaches and levels of efficiency, about which my colleagues know much more than I do, but the formula is simple enough – if the wind blows, you will produce electricity.
But things aren’t so easy when we start talking about biofuels, because there are many different kinds of biological raw materials.
They can all be transformed using a variety of methods that produce fuels of different quality. For this reason it’s a good idea first to define what we mean by quality in connection with biofuels.
- Firstly, how “useful” is the fuel for the engine in question?
- Secondly, how sustainable is the fuel?
Relevant value chains exhibit many differences, and many factors have to be taken into account when assessing the sustainability of a biofuel.
Resources such as water and fertilisers are needed to cultivate the biomass, and biomass harvesting may have a local impact on biological diversity and contribute towards deforestation. At the same time, biofuel production may bring great benefits simply because it replaces fossil fuels. Or the biofuel may help to resolve a waste problem. We often produce biofuels from waste raw materials – a subject that I have blogged about before.
Best generation
Firstly, we talk about biofuels in terms of generations:
- First generation biofuels are commonly derived from food plants, such as biodiesel from oilseed rape and bioethanol from sugar.
- Second generation biofuels are produced from lignocellulose materials derived from whole or parts of plants and trees that are not used for human consumption. These include all biofuels derived from wood, and include lignocellulosic bioethanol and synthetic diesel produced by means of gasification or the Fischer-Tropsch process.
- Third generation biofuels are those derived from aquatic biomass.
- Fourth generation biofuels are so-called “electrofuels”, and include the use of renewable electricity and carbon dioxide as feedstock. Such fuels occupy the margins of the definition of biofuels.
A good rule of thumb for biofuels is that the higher the generation, the more sustainable they are.
But there are exceptions. If when harvesting timber you exceed the volume that is sustainable for the forest in question (sustainable yield), the fuel produced will not have had an especially positive impact on the environment. At the same time, there are situations in which the combined cultivation of food and first generation biofuel raw materials is a stabilising factor for local economies, with benefits not only for the environment, but also for local communities.
How advanced is the biofuel?
Another term used is “advanced biofuel”. A biofuel can be defined as advanced for different reasons.
- One is based on the raw material. Here, an advanced biofuel corresponds to second, third or fourth generation biofuels. An example of this is biodiesel (containing fatty acid methyl esters) derived from recycled frying oil, which is by definition waste.
- A second way of defining an advanced biofuel is based on the technology used in its production. The term is applied to more costly and demanding technologies, such as the production of bioethanol from lignocellulose, or gasification followed by the Fischer-Tropsch process.
- A third definition is dependent on whether the fuel itself can be described as being of at least the same or better quality than its fossil equivalent. These are often called “drop-in” biofuels, meaning that they can be used to replace fossil fuels in existing infrastructures and engines without restrictions.
An example of this third definition depends on whether we modify the production technology and instead of biodiesel (ester), we produce HVO (hydrotreated vegetable oil). HVO is of at least the same or better quality than today’s diesel fuel and can be produced from waste fats or recycled frying oil, or cooking oils such as rape oil or palm oil. In this example, the fuels themselves are identical. However, since different raw materials are used, they have contrasting impacts on the environment.
Biofuels in Europe
EU legislation has adopted a definition originally developed by the European Industrial Bioenergy Initiative (EIBI, now a part of the European Technology and Innovation Platform, ETIP Bioenergy), which is attempting to govern most aspects concerned with ensuring that sustainable production can be maintained:
“Advanced Biofuels are those (1) produced from lignocellulosic feedstocks (i.e. agricultural and forestry residues, e.g. wheat straw/corn stover/bagasse, wood based biomass), non-food crops (i.e. grasses, miscanthus, algae), or industrial waste and residue streams, (2) having low CO2 emission or high GHG reduction, and (3) reaching zero or low ILUC impact”
In order for a biofuel to be described as advanced, it must be derived from a sustainable raw material, meet certain requirements regarding CO2 emissions reductions, and not contribute either directly or indirectly to changes in land use.
It is clear that the biofuel has to be sustainable. The EU requires documentation that all biofuels from existing refineries deliver greenhouse emissions reductions of at least 50% (2017), rising to 60% for new biofuel production facilities from 2018. But how do we know that these requirements are being met?
How do we measure sustainability?
Sustainability can be measured using an approach called Life Cycle Assessment (LCA). When we evaluate the environmental impact of a given value chain, assessments are made of the various stages during both the production and use of the biofuel. Following this, assessments are made of the resources and manpower used at each stage throughout the life cycle.
In the case of a biofuel, the factors we examine usually include the production and transport of the raw materials, the production and use of the fuel itself, and the environmental impact of sidestreams or waste. For example, it may be possible to measure the consequences of waste disposal from the production process.
Unfortunately, we find that even if standards have been defined for a given value chain, the measurements for such chains can vary considerably. This is because they operate with different system limits and references. For this reason it is important to adopt a coherent system when making life cycle assessments for biofuels.
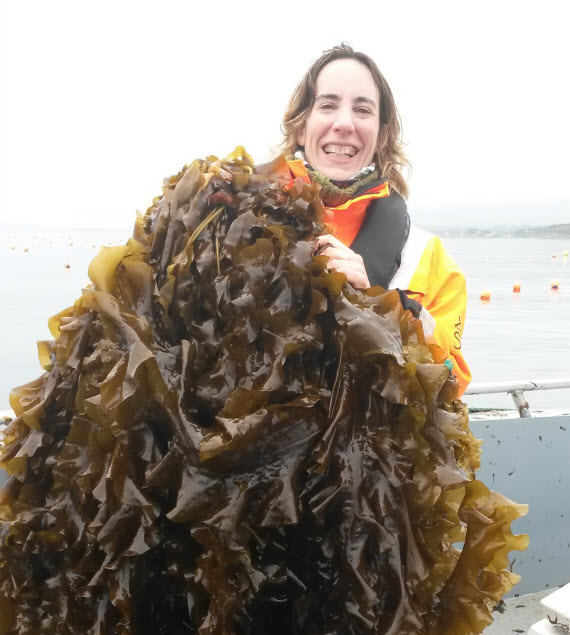
I have been working with various biofuel production processes (including those involving seaweed biomass) since the early 2000s. I also have a seat on the ETIP Bioenergy Steering Committee.
Biofuels are made up of the following molecules:
Petroleum-based fuels consist of hydrocarbons with different chain lengths:
Petrol: C4-C12
Jet: C12-C16
Diesel: C15-C18
The different fractions are obtained mainly from the distillation of crude oil.
HVO and FT diesel biofuels consist of exactly the same molecules as petroleum-based diesel.


Biodiesel contains additional oxygen (an ester functional group) in its carbon chain.

Bioethanol is made up of ethyl alcohol and is used as a fuel either in its pure form, or mixed with petrol:
There are also a number of more exotic biofuel molecules such as isobutanol and SIP (fermented hydroprocessed sugar):
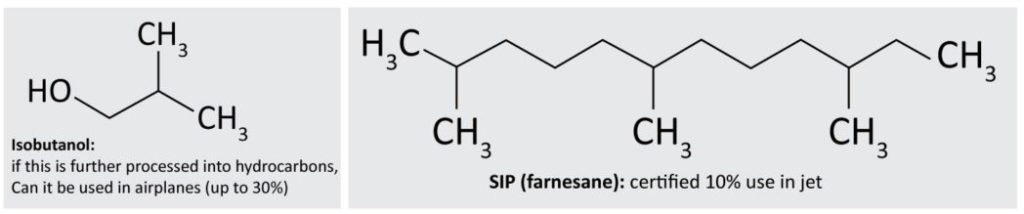
Biofuels are now, and will be in the future, an important contributor towards decarbonisation of the transport sector. It is important that the public authorities and consumers keep up with developments to ensure that sustainability is maintained at all levels.
We are working together to achieve the sustainable production and use of biofuels. If you want to find out more, visit the website Norges «biodrivstofflandslag» i Bio4Fuels senteret (Norway’s “biofuel team” at the Bio4Fuels Centre).
Comments
Biofuel has always been an interesting subject and topic of debate. Even in today’s time after such wide sources of knowledge, people are still unaware of the countless advantages of biofuels. For instance, many experts have stated that biodiesel is the fuel of the future. However, people still choose fuels that are hazardous to the environment. Do you know every day we contribute to air pollution and water pollution? We consistently pour the used cooking oil into the drain. It not only contaminates other water bodies but also harms marine life. It’s high time people should be aware that rather than just pouring and throwing away used cooking oil, they can donate it to Aris Bioenergy or any other biodiesel manufacturers.
In the internal combustion engine (ICEs) biobutanol has proved to be a superior synthetic biofuel. Fermentation engineering with acetone-butanol-ethanol (ABE) is a common technique used in biobutanol processing. However, its large-scale implementation has been obstructed by the high costs and extra energy use in the biobutanol recovery phase from intermediate fermentation solvent (i.e., ABE mixture). Investigating ABE as a possible renewable biofuel is getting more coverage. ABE development and ABE combustion have been extensively studied in ICEs, but so far these studies are rarely checked to support understanding and popularization of ABE. The updated progress of the ABE fermentation techniques is first summarized in this work from the following aspects: I selection of suitable strain; (ii) availability of cheaper substrates; (iii) production of fermentation engineering. The study on ABE combustion in ICEs is then derived from the following aspects: I physicochemical properties and testing of ABE components in ICEs; (ii) diesel substitution in compression ignition engines; and (iii) gasoline substitution in spark ignition engines. These studies indicate that ABE is a safer substitute for gasoline or diesel fuel due to the environmentally friendly production process and the potential for increasing energy quality and reducing pollutant emissions. However, ABE has not been intensively studied in contrast with traditional renewable fuels ( e.g. ethanol, butanol, biodiesel, etc.), for which there are large numbers of studies. Therefore, in the end, some problems and future directions for study are highlighted. This study is useful for identifying prospects in the near future to make ABE a viable renewable biofuel. Detail study can be found here https://www.sciencedirect.com/science/article/pii/S037838201930966X
This was very informative and helpful thanks for sharing.I have learned a lot by your article and http://asaagreenstation.com/